What is Schrodinger's Cat?
Schrodinger's Cat is a thought experiment created in 1935 by a brilliant scientist who loved quantum physics and hated cats.
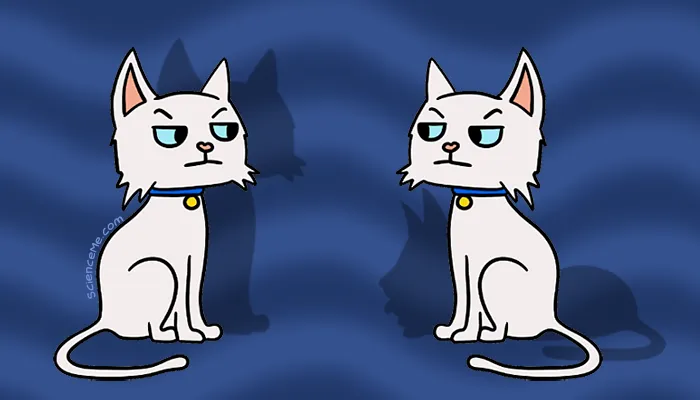
Inappropriately famous for his cat, Schrodinger was actually a science boss who was awarded a Nobel Prize for his work on wave mechanics. He also thrust physics onto biologists in his highly influential book, What is Life? But you're here for Schrodinger's cat, so let's spiral in on the ludicrous thought experiment devised to poke holes in the Copenhagen Interpretation of quantum theory.
Classical Physics vs Quantum Physics
Our story begins with the deeply unsettling clash between the worlds of classical physics and quantum physics.
In 1687, Newton gave us three laws of motion, proving that we live in a clockwork universe. We learned the physical world is entirely predictable with all events tightly bound by cause and effect, and is therefore deterministic. The Moon's orbit is deterministic because forces like gravity and inertia drive its position in space. With the relevant data we can predict exactly where the moon will go.
Causal determinism says there's an unbroken chain of events that goes all the way back to the Big Bang, and all the way forward to the end of the universe. Everything is determined because everything follows predictable laws.
So far, so good. But after Planck kicked-off the study of quantum physics in 1900, scientists arrived at a very different conclusion. In astounding conflict with Newton's clockwork universe, we learned that subatomic particles are probabilistic and therefore random. A dot is not a fixed point in space, but rather a theoretical wave of possible dots in space.
Do you see the problem? The Moon is a deterministic object made of regolith, bedrock, and a molten cheese core (I like to think). But it's fundamentally made of probabilistic quantum particles acting on random whims. For the Moon to behave as it does, we might also expect a crowd of insane looters to form an orderly queue and have their credit cards ready.
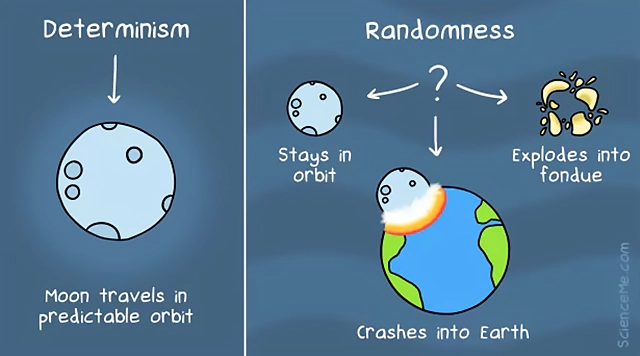
The classical world is causally determined; it could never be probabilistic and random.
How is this possible? Is everything Newton said suddenly wrong? No! Satellites won't fall out of orbit, bridges won't suddenly collapse, and seat belts won't inexplicably fail. But quantum mechanics isn't wrong, either: quantum technologies are ticking along just fine, from atomic clocks, to quantum cryptography, to entanglement-enhanced microscopes. The apparent conflict between these two domains just means that size does matter—and there are unknown factors we don't yet understand.
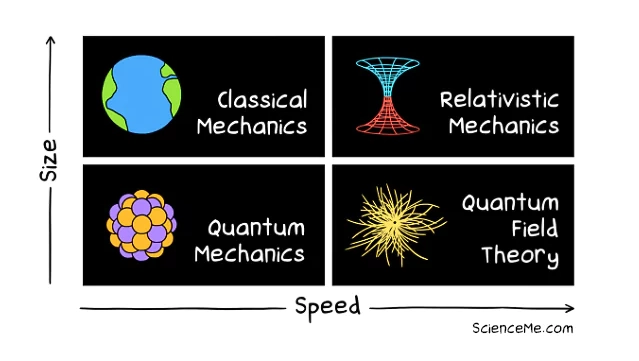
Schrodinger's Cat is a thought experiment that expresses this weirdness in a specific scenario. Still, the fact remains that the quantum and classical worlds have fundamentally conflicting playbooks, with the transition between the two depending on the scale, complexity, and environmental interactions of the particles involved.
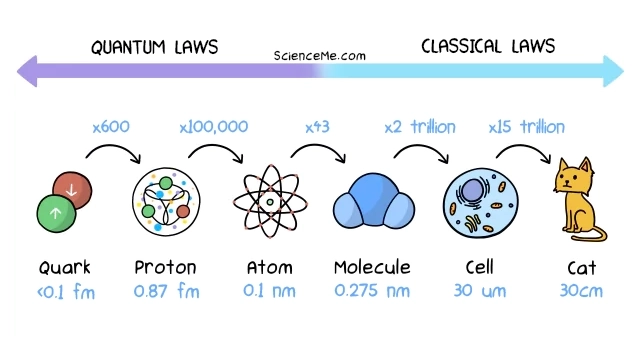
Now let's mix things up even more, because quantum particles aren't always wave-like in their behaviour. Sometimes they can behave like predictable particles. And it all seems to hinge on whether or not we're measuring them.
The Double Slit Experiment
Here's a concrete example where we can see light behaving predictably or randomly based on what's occurring in its environment. Here's what you do:
- Fire individual photons of light at a barrier with two slits
- Measure the photons with polarisers as they pass through one or other slit
- Observe two lines as the photons accrue on the detector screen
This is an intuitive result if we think of photons as discrete units. If you threw 100,000 darts at the slits, a bunch would bounce off the barrier, but those that got through would form the same double slit pattern.
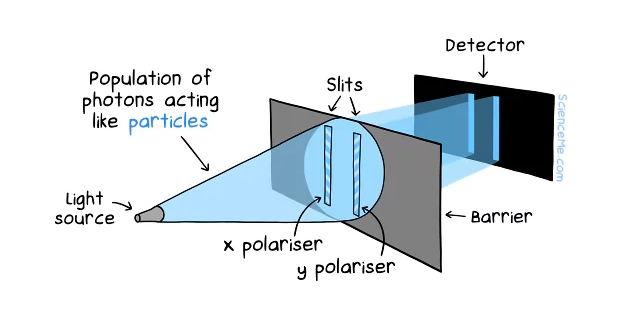
The Double Slit experiment shows populations of photons behaving like particles.
Next, you tweak just one variable: remove the polarisers so you're no longer measuring the photons in transit. You're now only measuring them once they've reached their destination on the detector screen, and you won't know which slit they took to get there. So you switch on your photon gun, leave the room and grab a cup of coffee. This is what you see when you get back.
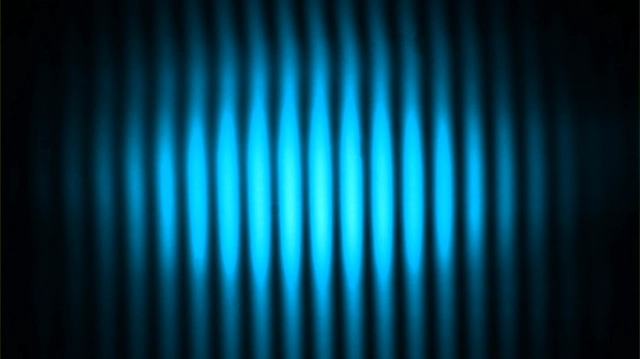
An interference pattern.
What's this? Who the devil has been messing with your experiment? The quantum overlords, that's who. Without measurement in transit, the photons of light switched from acting like deterministic particles to probabilistic waves. This phenomenon is called wave-particle duality.
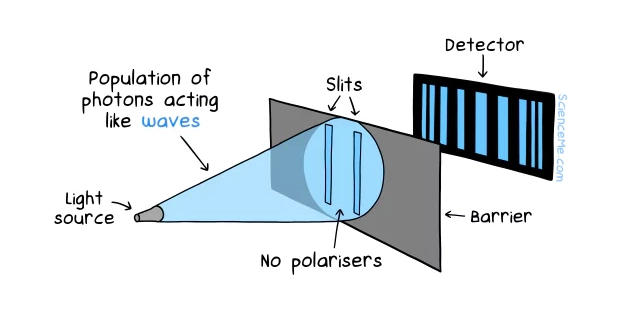
Wave-particle duality creates an interference pattern.
Quantum theory says that each photon travels as a wave of probabilities, embodying all possible routes to the detector screen. What's more, the waves interact with themselves, combining to form peaks or to cancel each other out, ultimately creating an interference pattern.
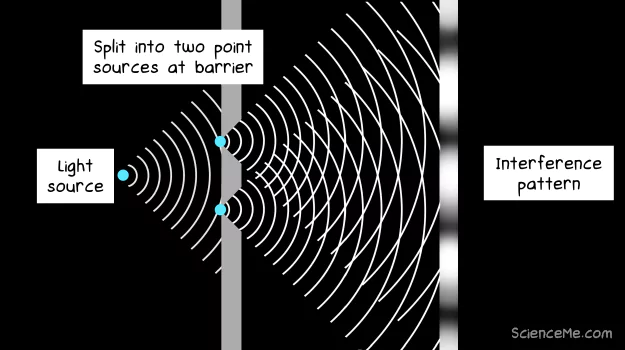
Quantum randomness creates interference patterns.
So far, so crazy. But why does light only play with itself when no-one's looking?
The Uncertainty Principle
The best explanation for the Double Slit experiment is known as the measurement-disturbance effect. There are various ways to measure single photons in real-time, like photo-detectors, photomultipliers, or single-photon detectors. However, any kind of measurement device inherently disturbs the photons in transit.
At the quantum scale, taking a measurement means bouncing other quanta off your target. This gives you data on a photon's position, but in doing so imparts energy that changes its momentum. This brings us to Heisenberg.
Heisenberg's Uncertainty Principle says it's impossible to know both the position and momentum of a quantum particle at the same time. By measuring one property, we inadvertently influence the other.
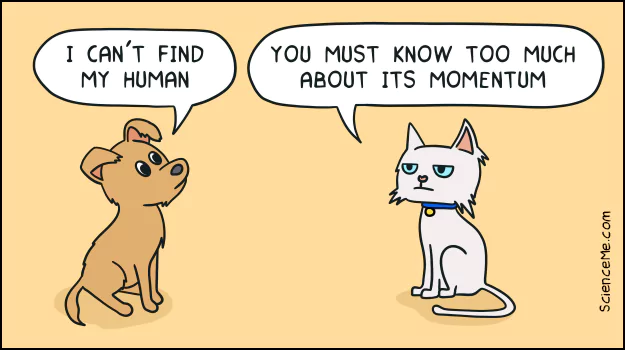
Uncertainty at the classical scale.
The observer effect refers to the act of measuring or observing a quantum system fundamentally changing the system itself. Even when you look at something, your eyes and brain are part of a complex system that interacts with the light reflecting off the object. In Dark Matter, Jason Dessen doesn't need the neurological compound to alter his consciousness; he needs to close his eyes and eliminate his physical presence to avoid interacting with the quantum system itself.
Panic over, right? The observer effect is just an artefact! Unfortunately, there's still a gap in the science. To date, experiments have found that the measurement-disturbance effect explains only half of the disturbance predicted by the Uncertainty Principle. There is still a mystery factor directing the quantum world.
The Copenhagen Interpretation
In the 1920s, physics heavyweights Bohr, Heisenberg, and Born came up with the Copenhagen Interpretation to explain the quantum world.
The Copenhagen Interpretation says that quanta exist in superposition of all possible states at once, described probabilistically by the wavefunction. Observation forces the wavefunction to collapse into a definite, deterministic state.
Collapse theories hold that quantum particles exist in probabilistic superposition. But there is debate about what causes the wavefunction collapse: Heisenberg believed it was something outside the quantum system, while Bohr said the collapse was a local process.
Einstein agreed with the mathematical framework but rejected the idea that reality depends on observation. He proposed hidden variables to explain quantum phenomena.
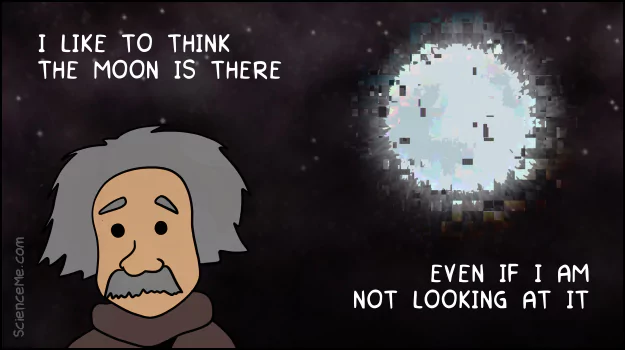
Like Schrodinger, Einstein was disturbed by the incompleteness of the Copenhagen Interpretation.
Heisenberg's need for an outside observer raises more questions. How did the universe form without any observation? Is objective collapse sometimes possible?
Whichever angle you take, the Copenhagen Interpretation gained widespread acceptance in the 1930s, and while Einstein's concerns were never fully addressed, it remains the most commonly taught view of quantum mechanics today.
The Many-Worlds Interpretation
Later, Everett pushed back with an alternative explanation and he only had to invent infinite universes to do so.
The Many-Worlds Interpretation says that quantum superpositions are objectively real. There is no wavefunction collapse because all quantum probabilities are realised in alternate universes.
Everett imagined a universal wavefunction that governs all possible realities. As superpositions break down, they separate into distinct universes and continue to exist independently.
The Many-Worlds interpretation implies you already died an infinite number of times before breakfast. Every possible scenario plays out in every moment of your life, such that your proctologist is a famous musician in other material realms, and vice versa.
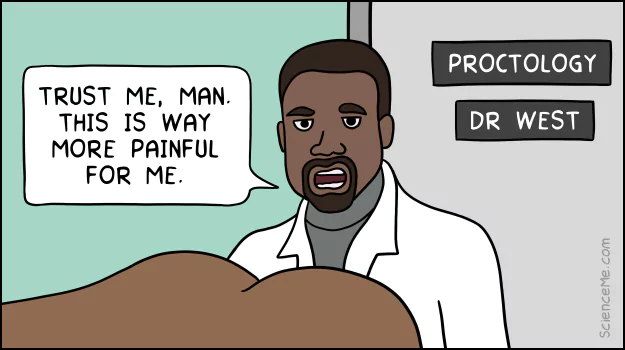
I have no idea how to caption this.
The Many-Worlds Interpretation cures us of the wavefunction collapse. It's a deterministic theory for a physical universe that also explains why the world can seem indeterministic, even if it does raise the new problem of infinite parallel worlds.
Quantum Entanglement
I promise Schrodinger's Cat is coming. But there is one more aspect of quantum theory causing physicists to cry themselves to sleep at night. It's called quantum entanglement.
"Quantum entanglement is the characteristic trait of quantum mechanics, the one that enforces its entire departure from classical lines of thought." - Erwin Schrodinger
Amid the quantum hullabaloo of the 1930s, Einstein, Podolsky, and Rosen published the EPR Paradox. Their thought experiment was designed to show how quantum theory was still terribly silly and must therefore be incomplete.
The EPR Paradox shows that, despite the uncertainty principle, quantum theory still allows us to measure the state of a photon without directly disturbing it. How? By taking the measurement from its entangled twin that lives very far away.
Quantum entanglement occurs when two or more quantum particles come into close proximity and take on a shared wavefunction. This invisible link allows particles to be correlated in their properties, such as spin or polarisation, even when separated by vast distances.
Einstein hated this idea because it violated the local realism view of determinism. He derided it as "spooky action at a distance".
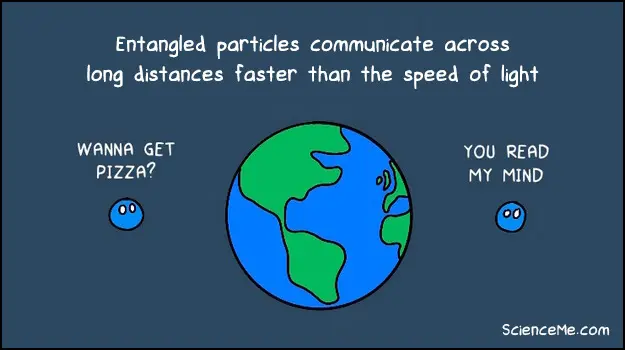
Quantum entanglement violates Einstein's idea that nothing can travel faster than the speed of light, including the simultaneous exchange of dinner plans between photons on different sides of the planet.
When Schrodinger read about the EPR Paradox, he wrote to Einstein suggesting the phrase quantum entanglement. Both agreed it was a crazy hypothetical implication of quantum theory. And yet it turned out to be entirely real.
Bell’s theorem showed that quantum entanglement can't be explained by local hidden variables. It demonstrated the non-local nature of quantum mechanics.
Bell inspired many experiments which proved quantum entanglement between photons, neutrinos, electrons, buckyballs, and even small diamonds. Indeed, entanglement now has practical applications in cryptography and microscopy, and work is underway to develop an ultrasecure quantum internet.
Schrodinger's Cat
I promised you a cat. And not just any cat, but one that's created and destroyed in order to undermine the Copenhagen Interpretation of quantum mechanics. In his thought experiment, Schrodinger imagined a closed system of:
- A radioactive atom with a 50:50 probability of decaying within the hour
- A Geiger counter measuring the atom's radiation
- A hammer suspended over a flask of acid
- An unimpressed cat
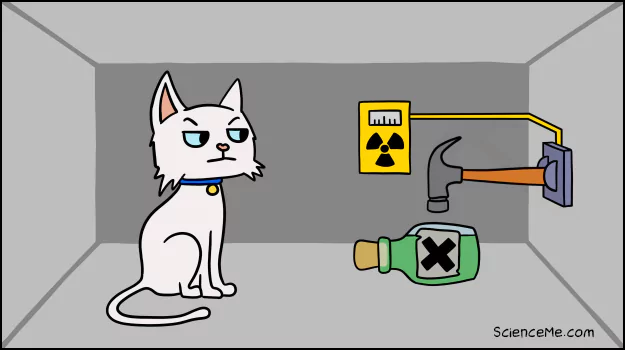
The initial conditions of Schrodinger's Cat.
Being a man of scientific rigour, Schrodinger's idea was to create a set of circumstances in which the cat's fate is entirely dependent on quantum probability. After one hour, there are two possible states for Schrodinger's Cat:
- He's alive. The atom didn't decay, which didn't trigger the hammer to fall, which didn't break open the flask of acid. The cat lives.
- He's dead. The atom did decay, which did trigger the hammer to fall, which did break open the flask of acid. The cat died. Sad face.
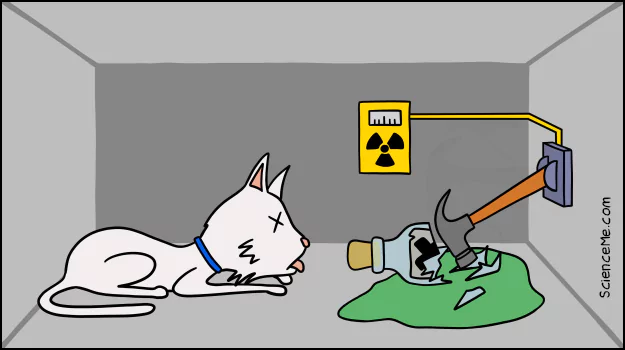
If the radioactive atom decays, Schrodinger's Cat is doomed.
Heisenberg's version of the Copenhagen Interpretation means that without an observer outside the quantum system, the cat's life hangs in the balance. The radioactive atom is suspended in a state of quantum superposition, taking the hammer, the acid, and the cat along for the ride. Schrodinger's Cat is neither dead nor alive. It exists in a blurry, non-real state until we open the box.
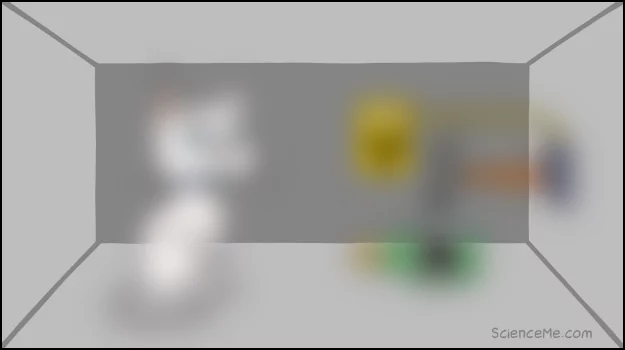
Schrodinger's Cat is in existential limbo in the Copenhagen Interpretation.
Schrodinger argued such an idea was naïve and ridiculous. Cats in life-threatening situations do not simply fall into limbo until we look at them and determine if they have become dead.
We all know it's nonsense to declare that something has become dead. It's just bad grammar. That's the whole problem with quantum theory, isn't it? It breaks all our comfortable rules. And Schrodinger thought it was all too much. So he called out Heisenberg. "This is bullshit," Schrodinger pointed out. Except he was Austrian, so he would have said: "Das ist Kuhscheiße."
Schrodinger wanted to illustrate how easy it is to arrive at absurd conclusions if we follow incomplete or inaccurate interpretations of quantum mechanics. It was ironic, then, when he admitted his own explanation would also "seem lunatic".
In 1952, Schrodinger made an early reference to the many worlds interpretation. He proposed that quantum superpositions are "not alternatives but all really happen simultaneously", inspiring Everett's formal Many-Worlds Interpretation a few years later.
Schrodinger and Everett treated the wavefunction as mathematical theory and physical reality. According to Many Worlds, the cat is alive in countless universes and dead in countless others; all possibilities actually occur as real events.
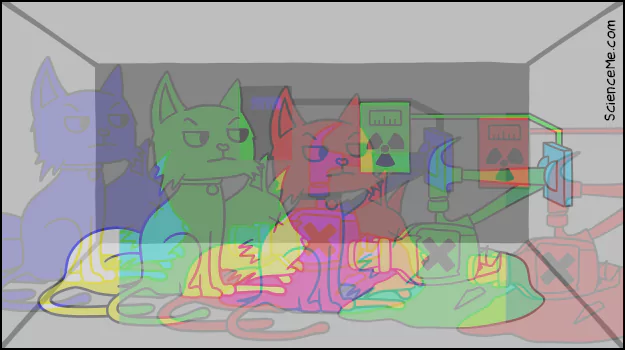
Schrodinger's Cat in the Many-Worlds Interpretation.
Confused? Disturbed? Horrified? Great. Welcome to the world of quantum theory.
Where does Schrodinger's Cat leave us? In practice, it turns out that it's very difficult to maintain quantum indeterminacy for tiny fractions of a second, let alone for an hour while we wait to seal our hypothetical cat's fate.
Also consider Bohr's idea: the wavefunction is collapsed by local interference. In this sense, the Geiger counter would interfere as a measurement device, and even the cat itself could be considered an observer of the system, thereby rendering the look-and-see scenario moot.
Modern physics has also introduced alternative explanations to the Copenhagen Interpretation, such as spontaneous collapse events imagined by the GRW Model. New ideas such as decoherence also explain how quantum systems transition from quantum to to classical behaviour.
So don't get too hung up on Schrodinger's Cat. It's a hypothetical thought experiment we can't actually test, and physicists are well over it. The rest of us mere mortals can use it as a weird and wonderful entry point into the incredible world of quantum physics.
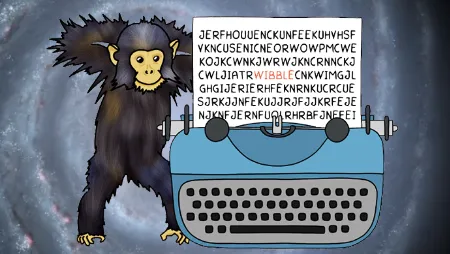
How Does Evolution Work?
Evolution connects all life on Earth; whether you're a marine worm or a marmoset, the same genetic code proliferates your DNA.
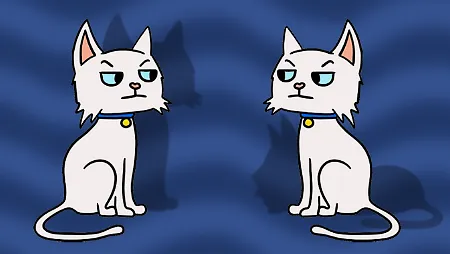
What is Schrodinger's Cat?
Schrodinger's Cat is a thought experiment created in 1935 by a brilliant scientist who loved quantum physics and hated cats.
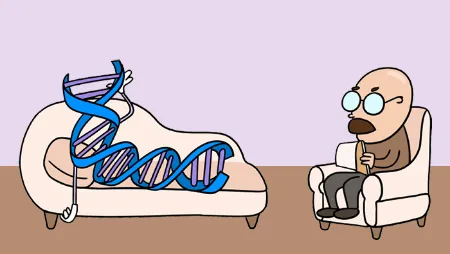
How Does Gene Therapy Work?
Gene therapy is a brilliant and terrifying emerging technology that uses viruses to deliver DNA payloads to faulty body cells to restore their function.
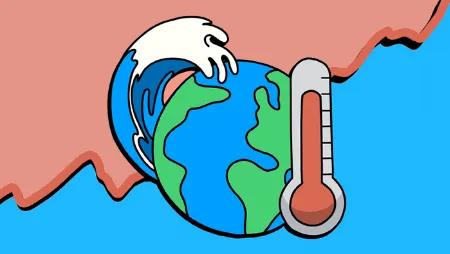
The State of Climate Change
An overview of natural and man-made climate change, including past, present, and forecast data on global temperatures and sea levels.
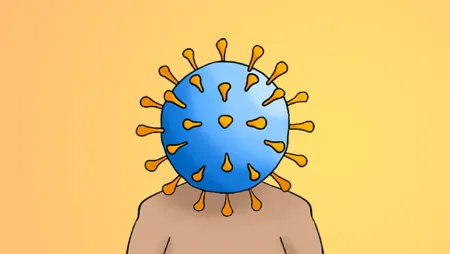
What COVID Does To Your Body
As the SARS-CoV-2 virus evolves, so too does the pathology of COVID, now classed as a vascular disease that can cause multi-system dysfunction.
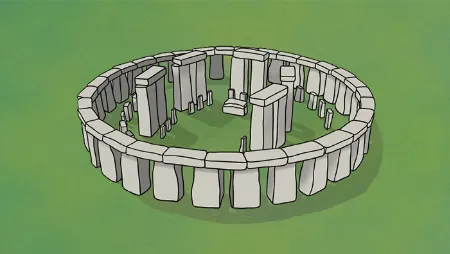
How Was Stonehenge Built?
In the 5,000 years since it was built, Stonehenge has been eroded by weather, sunken by earthworms, and picked at by handsy tourists.
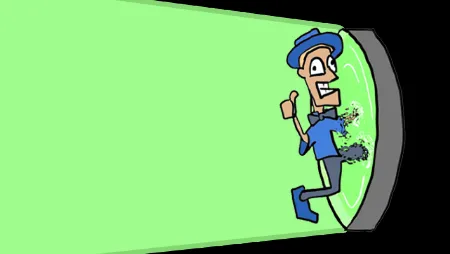
A Man Walks into a Teleporter
He pauses for thought while it scans every molecule in his body, makes a digital copy, and proceeds to annihilate his physical body.
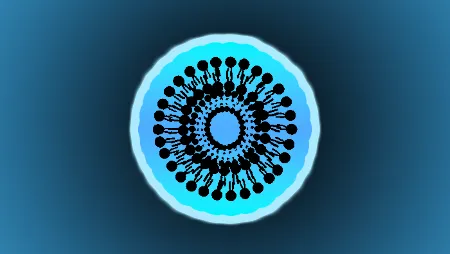
Nanomedicine is Here
Nanomedicine is shaping our lives, with 50+ nanomedicines already approved by the FDA, including nanoparticles in mRNA vaccines.
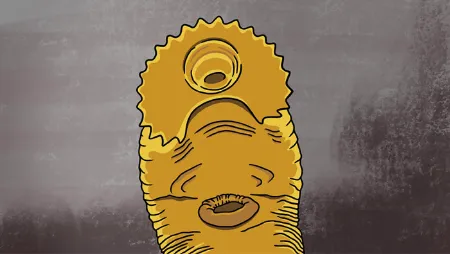
A Real Body-Snatching Parasite
Meet my associate, Curtuteria australis, a parasitic flatworm who takes three hosts during his convoluted lifecycle.
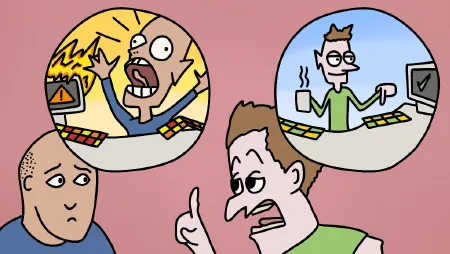
The Biology of Depression
Sapolsky says depression is a biochemical disorder with genetic and experiential influences which means you can't enjoy sunsets anymore.